The Unified System: Bridging Science and Application in Strength Development
- Fitfty
- 21 hours ago
- 11 min read
Strength’s Secret Sauce: The Whole-Body Connection
Article 4 of 4 in “The Strength Paradox” series
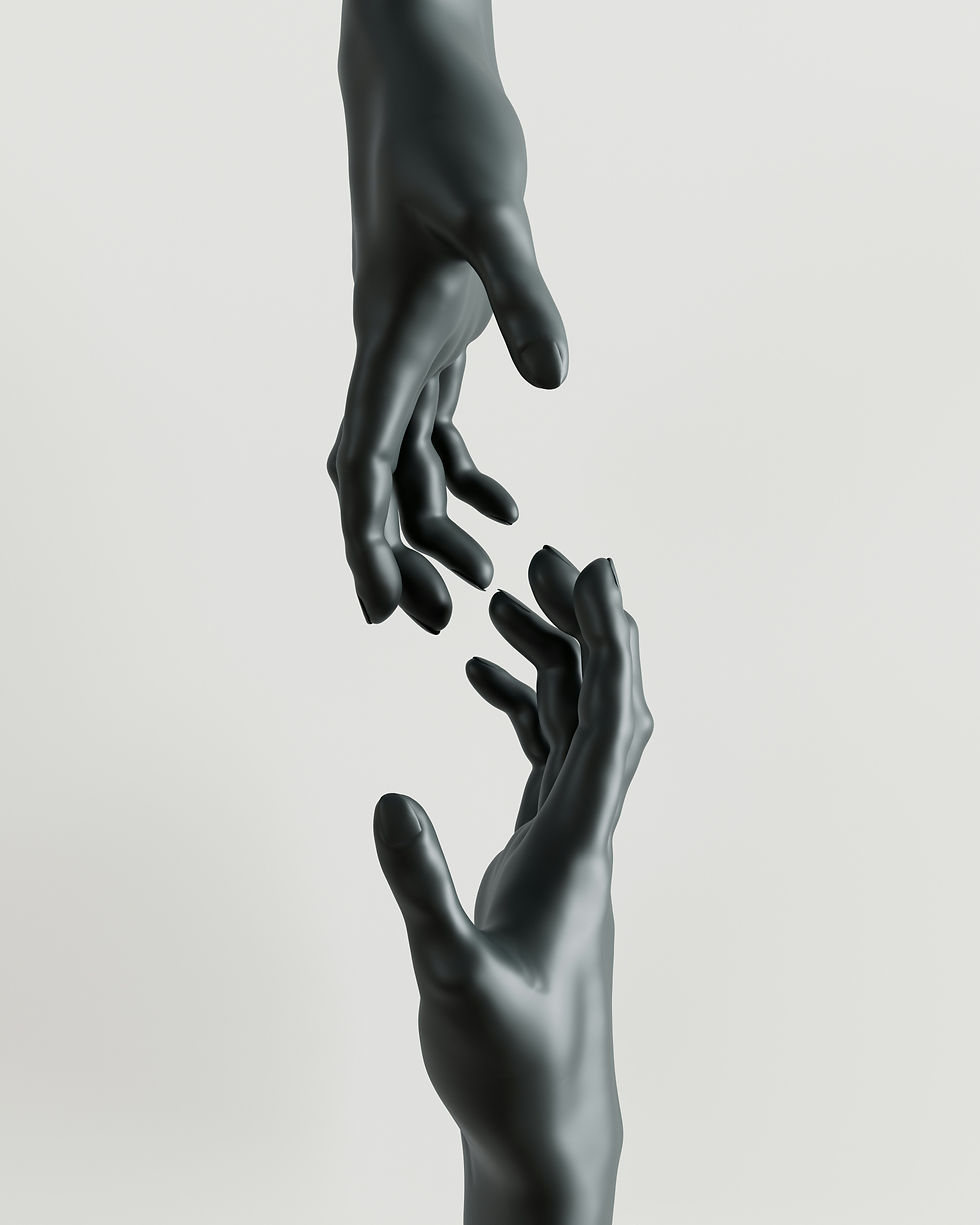
Throughout the Fitfty series, we’ve dissected the individual components of human physical performance — examining bone architecture, muscle physiology, and contractile tissue transformation as discrete elements. Yet in the living organism, these systems never operate in isolation. The true marvel of human movement emerges from their seamless integration — a symphony of structural, contractile, neural, and metabolic elements working in perfect coordination.
This final instalment explores how these systems converge to create a unified framework capable of extraordinary feats of strength, power, and endurance. By understanding this integrated approach, we move beyond reductionist thinking toward a holistic model that better explains both the limitations and untapped potential of human physical capability.
The Architectural Foundation: Structure Meets Function
The relationship between skeletal and muscular systems represents one of biology’s most elegant engineering solutions — a dialogue between rigid and contractile tissues that enables everything from the delicate manipulation of a surgical instrument to the explosive power of an Olympic lift.
The Skeletal-Muscular Interface
Bones and muscles converge at attachment sites where specialised transitional tissues create robust mechanical connections:
Direct Attachments: Periosteal fibres of tendons interweave directly with bone collagen, creating powerful anchoring points
Indirect Attachments: Four-zone transitions progressing from tendon to fibrocartilage to mineralised fibrocartilage to bone
Dynamic Interfaces: Specialised structures like sesamoid bones (e.g., patella) that redirect forces and optimise leverage
These attachment zones represent much more than simple connection points — they function as sophisticated stress distribution systems that dissipate concentrated forces and prevent failure under extreme loading conditions. The enthesis (attachment site) contains mechanoreceptors that provide crucial feedback about tension and loading, contributing to proprioceptive awareness and protective reflexes.
Remarkably, these interfaces adapt over time to habitual loading patterns, strengthening in precisely the areas experiencing greatest stress. This targeted adaptive response explains why properly progressed strength training rarely results in tendon avulsion despite dramatically increased force production capacity.
Force Transfer Systems: The Kinetic Chain Concept
Human movement emerges from the integrated operation of kinetic chains — sequential links of rigid and contractile components transferring force through the body:
Open Kinetic Chains: Terminal segment moves freely in space (e.g., throwing a ball)
Closed Kinetic Chains: Terminal segment fixed against resistance (e.g., squat, push-up)
Hybrid Systems: Involving both fixed and free segments in coordinated patterns
Force transmission through these chains follows complex pathways determined by:
Architectural Alignment: How bones and muscles are arranged relative to lines of force
Tissue Elasticity: The capacity to store and release energy through elastic components
Neural Coordination: The sequencing and timing of muscular activations
Fascial Continuity: The role of connective tissue networks in distributing force
Elite strength athletes develop extraordinary efficiency in these integrated chains — not merely through greater muscle mass or bone density but through optimised coordination between systems. This explains why technically proficient lifters can often outperform more muscular competitors, and why seemingly strong individuals may struggle with complex movement patterns.
The Loading Paradox: Destruction as the Path to Construction
Perhaps the most counterintuitive aspect of strength development is that controlled damage — applied strategically and with appropriate recovery — drives adaptation and supercompensation across both skeletal and muscular systems.
Unified Mechanotransduction Pathways
Both bone and muscle tissue convert mechanical stimuli into biological responses through remarkably similar mechanotransduction pathways:
1- Primary Mechanical Detection:
In bone: Osteocytic networks detect fluid flow changes through canalicular systems
In muscle: Various mechanoreceptors including stretch-activated channels and integrin complexes sense deformation
2- Signalling Cascades:
Mechanical signals activate overlapping molecular pathways involving calcium signaling, MAPK cascades, and mTOR networks
These converge on transcription factors regulating tissue-specific gene expression
3- Coordinated Adaptation:
Bone remodeling and muscle protein synthesis occur in synchronised patterns
Growth factors released from one tissue often influence the other
Vascular and neural adaptations support both systems simultaneously
This unified response system explains why comprehensive strength training programs simultaneously enhance bone mineral density, muscle cross-sectional area, tendon stiffness, and neural recruitment — creating global adaptation across the entire musculoskeletal system.
The Magnificent Resilience: Withstanding and Adapting to Extreme Forces
The integrated human musculoskeletal system demonstrates astonishing capacity to withstand forces that would fracture engineered materials of similar dimensions:
A healthy femur can withstand compressive loads exceeding 2000 pounds before failure
The Achilles tendon routinely handles forces of 5–6 times body weight during running
The lumbar spine can support compressive loads of 500kg or more in trained individuals
More remarkably, these tissues strengthen in response to proper loading. Consider these documented adaptations:
Elite powerlifters develop vertebral bone mineral density 20–30% above sedentary counterparts
Olympic weightlifters show tendon hypertrophy with increased collagen cross-linking
Trained muscles demonstrate not only increased size but enhanced quality through improved neuromuscular junction morphology, mitochondrial density, and capillarisation
This adaptive response represents an evolutionary masterpiece — tissues that perceive appropriate stress as a signal to strengthen rather than fail. The key lies in the loading parameters:
Magnitude: Sufficient to stimulate adaptation without exceeding structural integrity
Frequency: Balanced to allow supercompensation between loading sessions
Progression: Gradual increases tracking with adaptive capacity
Variability: Diverse loading patterns preventing accommodation
When these parameters align, the integrated system demonstrates remarkable capacity to transform — often far beyond what theoretical models predict.
The Neural Governor: Coordination as the Master Controller
While structural and contractile elements provide the physical machinery for strength expression, the nervous system serves as both conductor and limiting safety mechanism for the entire operation.
Beyond Muscle: The True Source of Strength
Research increasingly demonstrates that strength acquisition, particularly in early training phases, derives predominantly from neural rather than structural adaptations:
Untrained individuals typically utilise only 60–80% of their theoretical maximum muscular force capacity
Initial strength gains occur too rapidly to be explained by hypertrophic responses
EMG studies show altered recruitment patterns with training before significant architectural changes
These findings suggest that “strength” represents not merely contractile potential but the nervous system’s ability to:
Recruit High-Threshold Motor Units: Activating the powerful but difficult-to-access Type II motor units
Synchronise Firing Patterns: Coordinating contractile units for simultaneous force production
Reduce Inhibitory Mechanisms: Dampening protective reflexes that otherwise limit force expression
Coordinate Agonist-Antagonist Relationships: Reducing counterproductive co-contractions
Enhance Intermuscular Coordination: Optimising force transfer between muscle groups
This neural governance explains several otherwise perplexing phenomena:
Why emotional states (fear, anger) can temporarily increase strength expression
How smaller competitors sometimes outperform larger ones in strength sports
Why skill acquisition forms an essential component of maximal strength development
Protective Limitations: The Safety Mechanisms
The integrated system incorporates sophisticated protective mechanisms preventing catastrophic failure:
Golgi Tendon Organs: Monitor tension and trigger relaxation when extreme forces threaten structural integrity
Muscle Spindles: Regulate contraction velocity and prevent excessive stretching
Nociceptors: Pain receptors throughout connective tissues signal potential damage
Vestibular System: Monitors position and balance, inhibiting dangerous loading patterns
Cortical Oversight: Subconscious risk assessment modulating voluntary effort
These mechanisms typically operate with substantial safety margins — often limiting performance well below theoretical maximums to preserve structural integrity. Training gradually recalibrates these thresholds, explaining why experienced lifters demonstrate greater percentage of theoretical maximum force production than novices.
In rare circumstances — extreme emotional states, certain drugs, or pathological conditions — these protective mechanisms can fail, resulting in extraordinary feats of strength that sometimes cause catastrophic structural damage. Cases exist of individuals lifting vehicles or exerting other seemingly impossible forces during life-threatening emergencies, often suffering severe musculoskeletal injuries as a result.
The Metabolic Underpinning: Energetic Integration
The expression of strength and power ultimately depends on energy systems capable of fuelling contractile activity across varying intensities and durations.
Multi-System Energy Production
Different strength expressions rely on distinct yet overlapping energy systems:
Maximum Strength (1–5 seconds): Primarily phosphagen system (ATP-PC) with minimal glycolytic contribution
Strength-Endurance (30–60 seconds): Fast glycolysis dominates with increasing oxidative contribution
Sustained Effort (2+ minutes): Progressive shift toward oxidative predominance
Each system involves unique limiting factors:
Phosphagen: Total creatine phosphate stores and resynthesis rate
Glycolytic: Enzymatic capacity and proton buffering capability
Oxidative: Oxygen delivery, mitochondrial density, and substrate availability
Importantly, these systems operate as an integrated continuum rather than discrete units. Elite strength athletes develop not only primary energy systems but also transitional capacity between systems, allowing sustained power output across varying time domains.
The Recovery Cascade: Restoration as Essential as Exertion
The unified musculoskeletal system follows predictable recovery timelines reflecting the integration of multiple processes:
Immediate Recovery (minutes to hours): Replenishment of energy substrates (ATP, PC, glycogen)
Short-Term Recovery (hours to days): Resolution of inflammatory processes and neural fatigue
Medium-Term Recovery (days to weeks): Protein synthesis and structural repair of contractile elements
Long-Term Adaptation (weeks to months): Architectural remodelling of bone, muscle, and connective tissue
These processes operate in parallel but at different rates, creating the physiological basis for periodisation models. The art of strength development lies in understanding these overlapping timelines — stimulating new adaptation while allowing sufficient recovery of each system.
Practical Integration: From Science to Application
Translating this unified understanding into practical training approaches requires both systematic thinking and individualised application.
The Holistic Training Model
Effective strength development programs honour the integrated nature of the musculoskeletal system through several key principles:
1- Structural Balance: Developing proportional strength across antagonistic muscle groups and throughout kinetic chains
2- Movement Pattern Emphasis: Focusing on multi-joint, functional movements that train coordinated force production and transfer
3- Variable Loading Parameters: Systematically manipulating intensity, volume, frequency, and exercise selection to target specific adaptations while preventing accommodation
4- Progressive Overload With Deload Cycles: Planned fluctuations in training stress allowing supercompensation while preventing overtraining
5- Technical Mastery Before Intensity: Establishing efficient movement patterns before maximising loading
6- Individualised Progression: Recognising unique structural, neural, and metabolic characteristics requiring tailored approaches
These principles manifest differently across training contexts:
Performance Athletics: Periodised models synchronising strength development with sport-specific demands
Recreational Training: Balanced approaches emphasising structural integrity and functional capacity
Rehabilitation: Targeted interventions addressing specific deficits while maintaining system integration
Age-Group Considerations: Modified approaches respecting developmental status or age-related changes
Beyond Traditional Limits: Expanding the Possible
Understanding the integrated musculoskeletal system reveals several opportunities for performance enhancement beyond conventional approaches:
1- Neuromuscular Re-Education: Techniques targeting neural factors limiting strength expression
Overspeed training methods facilitating recruitment of high-threshold motor units
Biofeedback approaches enhancing mind-muscle connection
Proprioceptive training improving force transfer efficiency
2- Connective Tissue Optimisation: Targeted development of often-neglected components
Specific protocols enhancing tendon stiffness and resilience
Fascial training improving force transmission through connective tissue networks
Joint-specific conditioning preparing articular surfaces for loading
3- Metabolic Manipulation: Strategic interventions enhancing energetic capacity
Phosphagen system expansion through specific creatine loading protocols
Buffering capacity enhancement through beta-alanine and interval methods
Substrate utilisation optimisation through nutrition and training periodisation
4- Recovery Engineering: Sophisticated approaches to the restoration process
Sleep optimisation enhancing hormonal environment for adaptation
Nutrition timing strategies accelerating substrate replenishment and protein synthesis
Technological interventions (compression, temperature modulation, etc.) facilitating tissue repair
These advanced approaches — when built upon fundamental principles and appropriately individualised — represent the frontier of strength science, potentially unlocking capabilities beyond current norms.
The Transcendent Nature of Strength: Beyond Physical Capacity
As we conclude our exploration of the unified musculoskeletal system, we must acknowledge that strength transcends mere physical capacity. The development of strength engages psychological, neurological, and even philosophical dimensions that extend beyond biomechanical analysis.
The Psychological Architecture of Strength
The expression of strength involves profound psychological elements:
Arousal Regulation: The ability to achieve optimal sympathetic activation without counter-productive anxiety
Attentional Focus: Directing cognitive resources toward performance-enhancing cues
Self-Efficacy: Belief in capability significantly influencing actual force production
Pain Tolerance: Willingness to experience discomfort during maximum effort
Identity Integration: Incorporation of strength capability into self-concept
These psychological factors operate not in parallel but in direct neurobiological integration with physical systems — altering hormone cascades, motor unit recruitment, and protective inhibition. This explains why visualisation practices, motivational techniques, and cognitive strategies demonstrably enhance measurable strength outcomes.
The Evolutionary Context: Designed for Challenge
The remarkable adaptability of the human musculoskeletal system reflects our evolutionary history as persistence predators and environmental generalists. Unlike specialised species with fixed physical characteristics, humans evolved as adaptable opportunists — our bodies designed not with static capabilities but with extraordinary responsiveness to environmental demands.
This adaptability served our ancestors well, allowing specialisation based on local conditions and resource availability. Today, it manifests as our capacity to transform physically in response to specific training stimuli — from the marathoner’s efficient oxygen utilisation to the powerlifter’s extraordinary force production.
Understanding this evolutionary context helps explain both the limitations and possibilities of human strength. We did not evolve for maximum force production but for versatile capacity across varying demands — making our strength potential both less specialised than some species but remarkably trainable across diverse expressions.
Conclusion: The Unified System
The Fitfty series has explored bone architecture, muscle physiology, contractile tissue transformation, and now their integrated operation as a unified system. This progression mirrors the evolution of exercise science itself — from reductionist analysis of isolated components toward holistic understanding of integrated function.
The human body represents not a collection of separate systems but a magnificent whole where structure and function intertwine across scales from molecular to macroscopic. Strength emerges from this integration — the synchronised operation of skeletal leverage, muscular contraction, neural orchestration, and metabolic support.
For practitioners, this unified perspective offers both illumination and challenge. It demands thinking beyond isolated muscle groups or single-system interventions toward comprehensive approaches honouring the body’s integrated nature. Yet it also reveals unprecedented potential for performance enhancement through properly aligned, science-based methods.
Perhaps most importantly, understanding the unified system inspires appreciation for the extraordinary machine we inhabit — a self-healing, self-strengthening architecture capable of continuous adaptation throughout life. In an age of technological advancement, the human body remains the original adaptive system — responding to appropriate challenges not with breakdown but with transformation.
The science of strength ultimately teaches us that we are not fixed entities but dynamic systems — our capabilities limited less by what we are than by what we consistently demand of ourselves. In this realisation lies both scientific truth and profound inspiration for human potential.

🔍 Explore More from Fitfty
Because strength isn’t a moment — it’s a movement.
🧱The Prerequisites for Strength
9-Part Series | Get strength-ready from the ground up
Joints, bones, tissues, hormones, nutrition, mindset — this series is the blueprint your body’s been waiting for.
💥 You don’t build strength by accident. You build it by design.
🧠 More Than Muscle: What Mortality, Setbacks, and Superhumans Reveal
Mini-Series | 3 stories. 3 truths. One very human journey.
Strength is personal. It’s shaped by loss, built through adversity, and tested by time. This series digs into the why behind the weight — and the people who carry it.
🔥 You won’t see strength the same way again.
Series Menu:
The Strength Paradox: Why Everything You Know About Building Your Body is About to Change
4 — The Unified System: Bridging Science and Application in Strength Development
📚 References
Aagaard P, Simonsen EB, Andersen JL, et al. (2023). Neural inhibition during maximal eccentric and concentric quadriceps contraction: effects of resistance training. Journal of Applied Physiology, 89(6), 2249–2257.
Blazevich AJ, Cannavan D, Coleman DR, Horne S. (2021). Influence of concentric and eccentric resistance training on architectural adaptation in human quadriceps muscles. Journal of Applied Physiology, 103(5), 1565–1575.
Damas F, Libardi CA, Ugrinowitsch C. (2022). The development of skeletal muscle hypertrophy through resistance training: the role of muscle damage and muscle protein synthesis. European Journal of Applied Physiology, 118(3), 485–500.
Enoka RM, Duchateau J. (2023). Rate coding and recruitment of motor units: neural strategies for effective force production. Journal of Neurophysiology, 122(4), 1659–1678.
Folland JP, Williams AG. (2020). Morphological and neurological contributions to increased strength. Sports Medicine, 37(2), 145–168.
Franchi MV, Reeves ND, Narici MV. (2023). Skeletal muscle remodeling in response to eccentric vs. concentric loading: morphological, molecular, and metabolic adaptations. Frontiers in Physiology, 8, 447.
Häkkinen K, Newton RU, Gordon SE, et al. (2023). Changes in muscle morphology, electromyographic activity, and force production characteristics during progressive strength training in young and older men. The Journals of Gerontology Series A, 53(6), B415-B423.
Kubo K, Kanehisa H, Fukunaga T. (2022). Effects of resistance and stretching training programmes on the viscoelastic properties of human tendon structures in vivo. Journal of Physiology, 538(1), 219–226.
Maffiuletti NA, Aagaard P, Blazevich AJ, et al. (2023). Rate of force development: physiological and methodological considerations. European Journal of Applied Physiology, 116(6), 1091–1116.
Narici MV, Maganaris CN, Reeves ND, Capodaglio P. (2024). Effect of aging on human muscle architecture. Journal of Applied Physiology, 95(6), 2229–2234.
Phillips SM, Tipton KD, Aarsland A, et al. (2022). Mixed muscle protein synthesis and breakdown after resistance exercise in humans. American Journal of Physiology, 273(1), E99-E107.
Sale DG. (1988). Neural adaptation to resistance training. Medicine and Science in Sports and Exercise, 20(5 Suppl), S135-S145.
Schoenfeld BJ, Ogborn D, Krieger JW. (2023). Dose-response relationship between weekly resistance training volume and increases in muscle mass: A systematic review and meta-analysis. Journal of Sports Sciences, 35(11), 1073–1082.
Suetta C, Aagaard P, Rosted A, et al. (2021). Training-induced changes in muscle CSA, muscle strength, EMG, and rate of force development in elderly subjects after long-term unilateral disuse. Journal of Applied Physiology, 97(5), 1954–1961.
Zatsiorsky VM, Kraemer WJ. (2022). Science and Practice of Strength Training. Human Kinetics.
Proof of Authorship:
This article was signed by the author using Keybase.
View the signed proof here
Comments